Gravity may be the weakest of the fundamental forces in nature, but it is ultimately what enabled life on Earth to evolve. Thanks to its weak attractiveness over long distances, mass in the early universe could clump together and form galaxies, stars and planets such as our own.
Our working theory for gravity comes from Albert Einstein’s general theory of relativity, which states that gravity is a consequence of massive objects warping the very fabric of spacetime. Scientists have validated the theory with great precision in our solar system, but we haven’t been able to do the same on larger distances – until now.
Our new study, published in Science, shows that general relativity also holds true on the scale of entire galaxies. The findings strengthen the popular view in cosmology that 95% of the universe is made up of invisible substances dubbed dark matter and dark energy – ruling out several competing theories.
The first ever test of general relativity was carried out by Arthur Eddington in 1919. As massive objects bend spacetime, light rays should be deflected as they pass the object rather than travelling in a straight line. Eddington managed to show that this was the case for light bending around the sun during a solar eclipse. Perhaps surprisingly, it has taken exactly 99 years for us to do the same for an entire galaxy.
Cosmic alignment
The galaxy we investigated has the catchy name ESO 325-G004 – let’s call it E325. Located some 450m light years away, it is one of the closest examples of a rare cosmic alignment – sitting directly between us and a second, more distant, galaxy. The background galaxy in this case is some 17 billion light years further behind. The centres of these two galaxies are aligned to better than one ten-thousandth of a degree.
Because light rays from the distant galaxy are deflected as they travel through the curved spacetime around E325, we see images of it that are slightly distorted from what we would otherwise see – an effect called gravitational lensing. It’s a bit like looking at an object through the stem of a wine glass. The deflection of light passing E325 is about 1/1200 of a degree.
If the curvature of spacetime near the first galaxy is great enough – and in the case of E325 it is – then multiple images of the background galaxy will form on either side of the lens galaxy when looking at it with a telescope. The image below shows this effect as we observed it with the Hubble Space Telescope. The size of this so-called “Einstein ring” tells us how much spatial curvature there is around E325 – more curvature means a bigger ring.
We also measured the amount of mass in E325 by looking at how fast the stars are moving in the galaxy. Similar to the Earth orbiting the sun, the stars in E325 orbit around the galaxy’s centre of mass, with gravity holding them in their trajectories. More mass in the galaxy means a stronger gravitational force and so the stars orbit faster.
To measure their speed, we used the “Doppler effect” – the stretching or squashing of waves because of motion. A radar speed camera makes use of it by detecting the change in the radio frequency from signals bounced off cars to measure their speed. In a similar way, we measured the change in frequency in the light from stars to estimate their speed. The light from stars moving towards us is slightly shifted to the blue (a specific frequency), and stars moving away are shifted to the red. The faster they move, the bigger the shift.
Because E325 is so distant, it’s not possible to measure the Doppler effect for individual stars. We instead measured the light from all the stars in a patch and estimated the different velocities using statistical methods. These observations were made using the Very Large Telescope in Chile.
Once we had measured the velocities of the stars and the radius of the “Einstein ring”, we combined the two results to see if the amount of spatial curvature was consistent with the total mass of the galaxy. We found that it was, with a mere 0.9% total uncertainty. This is the most precise test of general relativity over length scales larger than the solar system. Although scientists have made similar measurements previously, they have never managed to achieve the precision we did.
Dark energy and matter
But why should we care so much about whether Einstein was right or wrong? There’s actually a lot at stake – much of our cosmological understanding comes from interpretation of observations of the universe that depend on general relativity being correct. If general relativity didn’t hold up, cosmology would truly be in crisis.
According to most cosmologists, the vast majority of our universe is made up of dark matter and dark energy. Dark matter is needed to explain the observed motions of stars in galaxies. While we can’t see it directly, we can see that it has a gravitational pull on stars. Meanwhile, dark energy – which exerts an expansive force on the universe – is needed to explain the fact that the expansion of the universe is speeding up.
But there are alternative theories of gravity that can explain away these mysterious substances. They typically tweak how gravity works over long distances so that dark energy isn’t needed to explain the observations.
But our result poses a problem for these alternative theories by showing that gravity does behave the way general relativity expects on scales of up to 6,000 light years. Not only does our study therefore validate Einstein, it also shows that either dark energy and dark matter are real (whatever they are), or general relativity needs to be amended only on length scales that are larger than galaxies.
In the next decade, two new telescopes – the Euclid satellite and the Large Synoptic Survey Telescope – will be able to detect deviations from general relativity on scales more than 1,000 times longer than probed in E325. If general relativity also passes these tests we will know it is the right theory to describe gravity’s effects on the universe as a whole. So far, it is looking good for Einstein.
Thomas Collett, Research Fellow in Astrophysics, University of Portsmouth.
This article first appeared on The Conversation.
Scroll has produced award-winning journalism despite violent threats, falling ad revenues and rising costs. Support our work. Become a member today.
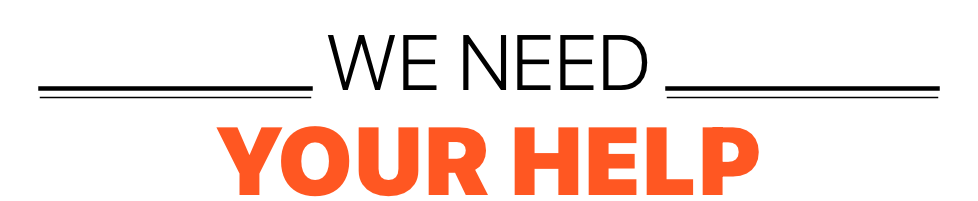
In these volatile times, Scroll remains steadfastly courageous, nuanced and comprehensive. Become a Scroll Member and support our award-winning reportage, commentary and culture writing.